Conventional NDT
Non-Damaging, Cost-Effective, Ease of Application
Conventional Nondestructive Testing (NDT) refers to a set of well-established inspection techniques used to evaluate materials, components, or systems for defects without causing any damage. These methods are widely applied across various industries due to their effectiveness in detecting common flaws and their cost-efficiency.
Have any question?
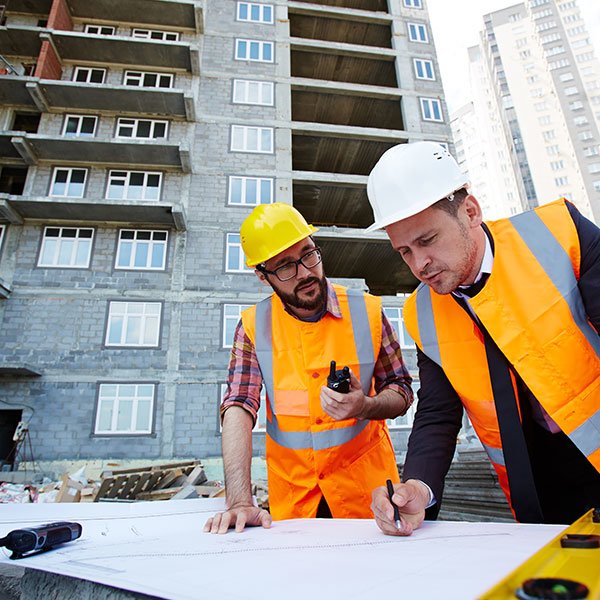
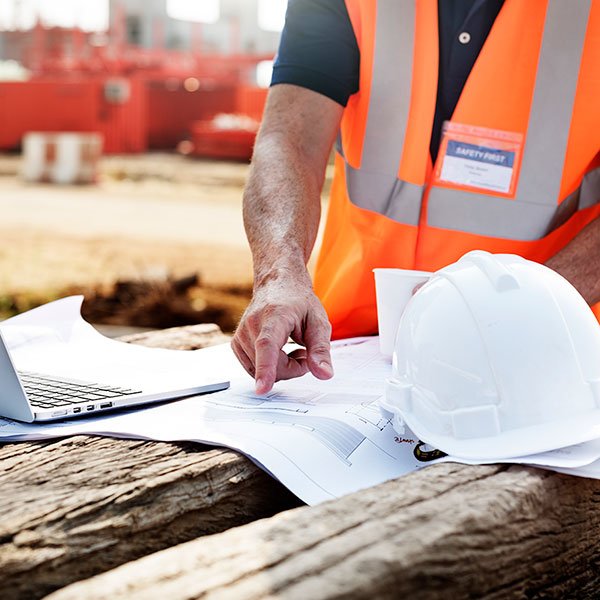
Visual Inspection (VT)
Visual Inspection (VT) is the oldest and most fundamental method of non-destructive testing (NDT).
It involves the direct observation of a component’s surface to identify any visible defects such as cracks, corrosion, misalignment, or other forms of damage. This technique is widely used across various industries due to its simplicity and effectiveness in detecting surface flaws.
Importance of Visual Inspection
Applications of Visual Inspection
Ensuring welds meet specified standards and identifying potential defects before they lead to failures.
Conducting inspections on tanks, pressure vessels, and process piping to ensure compliance with industry standards.
Monitoring manufacturing processes to maintain product integrity and safety.
Evaluating the condition of protective coatings on various surfaces.
Regular checks on lift/aerial equipment and other machinery to ensure operational safety.
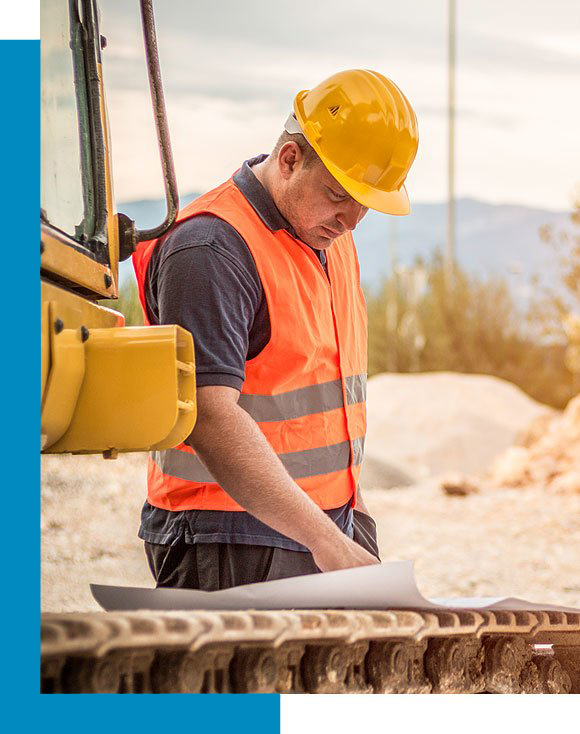
Ferrite Measurement
Ferrite measurement is a crucial aspect that evaluates the ferrite content in stainless steel and other alloys.
Several companies specialize in this service, providing accurate assessments to ensure material integrity and compliance with industry standards.
Importance of Ferrite Measurement
Methods of Ferrite Measurement
Ultrasonic Testing Shear wave (UT)
How Shear Wave Testing Works
Applications of Shear Wave UT
Shear wave UT is predominantly used in industries where welding is common, such as construction, manufacturing, and oil & gas sectors. It allows inspectors to evaluate weld quality effectively by identifying internal defects that could compromise structural integrity
This technique can also be adapted for corrosion mapping, helping assess material degradation over time due to environmental factors.
An advanced form of shear wave testing involves phased array technology, which uses multiple elements in a single probe to create detailed images of complex geometries. PAUT enhances detection capabilities by providing more precise control over beam angles and focusing.
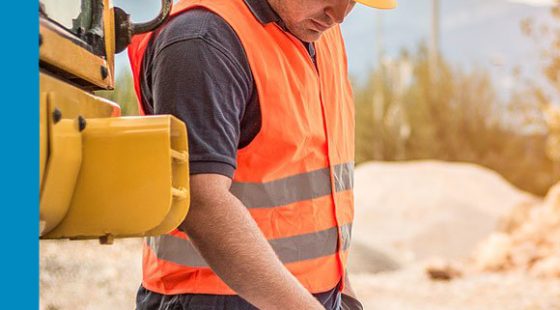
Liquid Penetrant Testing (LPT)
Liquid Penetrant Testing (LPT) also known as Dye Penetrant Testing (DPT), is a widely used method designed to detect surface-breaking flaws such as cracks, porosity, laps, and other discontinuities in non-porous materials.
This technique is particularly valued for its simplicity, cost-effectiveness, and ability to inspect large areas or complex geometries.
Principle of Liquid Penetrant Testing
The principle behind LPT relies on capillary action, where a liquid penetrant is drawn into surface-breaking defects. After applying the penetrant to the test surface and allowing it to dwell for a specified time, the excess penetrant is removed. A developer is then applied to draw out the trapped penetrant from defects, creating visible indications that can be inspected under appropriate lighting conditions.
Applications of Liquid Penetrant Testing
- Inspection of welds for cracks or porosity.
- Detection of casting defects like shrinkage cracks or cold shuts in foundries.
- Maintenance checks in aerospace industries (e.g., aircraft fleet maintenance).
- Examination of piping systems in nuclear power plants and other energy sectors.
- Quality control during manufacturing processes for automotive parts and tools.
Advantages of Liquid Penetrant Testing
- Can detect very small surface-breaking flaws (as narrow as 150 nanometers).
- Applicable to both metallic and non-metallic materials provided they are non-porous.
- Cost-effective with minimal equipment requirements compared to other NDT methods like radiographic testing or ultrasonic testing.
- Suitable for inspecting complex geometries and large areas quickly.
- Provides clear visual indications that help assess defect size and location.
Limitations of Liquid Penetrant Testing
- Only detects surface-breaking flaws; subsurface defects remain undetected.
- Not suitable for porous materials as they absorb penetrants indiscriminately.
- Requires thorough pre-cleaning; contaminants can interfere with results.
- Environmental factors like temperature can affect performance—optimal testing temperatures range between 27°C and 49°C.
Magnetic Particle Inspection (MPI)
Magnetic Particle Inspection (MPI) is a widely used method that detects surface and near-surface discontinuities in ferromagnetic materials. This technique is particularly effective for identifying defects such as cracks, laps, seams, and inclusions in materials like iron, nickel, cobalt, and their alloys. The following sections outline the principles, processes, equipment, advantages, limitations, and applications of (MPI).
Advantages of MPI
Applications of Magnetic Particle Inspection
- Aerospace components such as engine parts and landing gear.
- Automotive parts including axles and crankshafts.
- Structural steel inspections for bridges and buildings.
Oil and gas pipelines.
Limitations of MPI
MPI only works effectively on ferromagnetic materials such as iron and nickel; it cannot be used on non-ferrous metals like aluminum or magnesium.
After testing, components often need demagnetization to prevent interference with subsequent operations or electronic equipment.
While MPI can detect surface and near-surface flaws, it is not suitable for identifying deeper subsurface defects.
Ultrasonic Thickness Measurement (UTT)
Ultrasonic thickness measurement (UTT) is a widely used method that assesses the thickness of materials, primarily metals, by utilizing high-frequency sound waves. This technique is essential for evaluating the integrity and safety of various structures and components, such as pipelines, pressure vessels, and ship hulls.
Advantages of UTT
Non-destructive: It does not damage or alter the test piece during measurement.
Single-Sided Access: Measurements can be taken from one side only, making it suitable for inaccessible areas.
Applications of UTT
UTT is crucial for detecting corrosion or erosion in metal structures. By measuring remaining wall thickness, technicians can determine if maintenance or replacement is necessary.
It helps assess structural integrity in various applications including storage tanks, pipelines, and pressure vessels to ensure they can withstand operational conditions safely.
UTT can verify weld quality by measuring weld thickness against specified standards.
Regular inspections using UTT help organizations comply with safety regulations by identifying potential weaknesses before they lead to failures.
Limitations
For accurate measurements, surfaces may need cleaning or preparation to remove coatings or rust that could interfere with readings.
Each type of material requires specific calibration settings to ensure accuracy; improper calibration can lead to erroneous results.
Measurements may be less reliable on pitted or heavily corroded surfaces unless specialized equipment is used.
Positive Material Identification (PMI)
Importance of PMI
Regulatory Compliance: Many industries are subject to strict regulatory requirements regarding material use. Implementing PMI helps organizations comply with these regulations by ensuring that all components are made from appropriate materials.
Methods of PMI
Description: XRF is the most commonly used method for PMI due to its portability and ease of use. The handheld device emits X-rays onto the material being tested; the material then emits secondary X-rays that are characteristic of its elemental composition.
Advantages: It provides rapid results and can be used on-site without extensive preparation.
Limitations: While effective for many elements, XRF cannot detect lighter elements such as carbon, which limits its ability to differentiate between certain grades of stainless steel.
Description: OES involves creating a spark between an electrode and the sample material, exciting the atoms and causing them to emit light at specific wavelengths. This light is analyzed to determine the elemental composition.
Advantages: OES can detect almost all elements, including carbon and lighter elements, making it suitable for comprehensive analysis.
Limitations: The equipment tends to be larger and less portable than XRF devices, requiring more setup time.
Infrared Thermography
Principles of Infrared Thermography
Advantages of Thermography Techniques
Speed: Rapid inspection capabilities allow for quick assessments over large areas.
Real-time Results: Immediate feedback on material conditions helps facilitate timely decision-making regarding repairs or maintenance.
Applications
Aerospace Industry: It is extensively used for inspecting aircraft components for fatigue cracks, delamination’s in composites, and other structural integrity issues
Automotive Sector: Used for detecting faults in vehicle bodywork and ensuring quality control during manufacturing processes.
Types of Infrared Thermography Techniques
This method uses short bursts of high-intensity light (e.g., from flash lamps) to rapidly heat the surface of a test object. The subsequent cooling process is monitored using an infrared camera. Defects cause localized changes in cooling rates that can be detected.
n this technique, a periodic heating source is used to create a sinusoidal temperature variation on the surface. The phase shift between heating and response allows for enhanced detection sensitivity to subsurface defects
This combines ultrasonic excitation with thermal imaging. Ultrasonic waves generate localized heating at defect interfaces due to frictional effects, which can then be detected by infrared cameras.
A laser beam scans across the surface of a material, providing precise control over heating patterns and enabling effective detection of both horizontal and vertical cracks.
This method employs sinusoidal light patterns projected onto surfaces to create high-density thermal excitation for detecting fine defects in materials with high thermal conductivity.
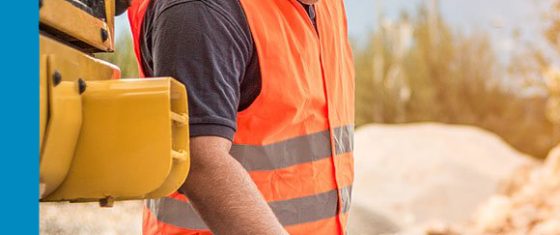
Industries: Commonly used in aerospace, automotive, electronics, and medical device manufacturing.
Radiography Testing X –Ray & Gamma Ray (RT)
Radiography Testing (RT) is a widely used method that employs X-rays or gamma rays to inspect the internal structure of materials, components, or assemblies without causing any damage. This technique is particularly effective for detecting internal defects such as cracks, voids, inclusions, and other discontinuities in welds, castings, and other materials.
Principles of Radiography Testing
X-Ray Radiography
Source
X-rays are generated by X-ray machines using high-voltage electricity to accelerate electrons toward a metal target. When these electrons collide with the target material, X-rays are produced.
Applications
Advantages
- High-quality imaging with excellent resolution.
- Adjustable energy levels allow customization for specific applications.
- Safer than gamma rays due to controlled production.
Limitations
- Limited penetration depth compared to gamma rays.
- Requires access to electricity and bulky equipment.
Gamma Ray Radiography
Source
Gamma rays are emitted from radioactive isotopes such as Iridium-192 (^192Ir), Cobalt-60 (^60Co), or Cesium-137 (^137Cs). These isotopes naturally emit high-energy photons during radioactive decay.
Applications
Advantages
- High penetration capability allows inspection of dense or thick materials.
- Portable sources enable field inspections without reliance on electrical power.
- Effective over long distances.
Limitations
- Lower image resolution compared to X-rays.
- Safety concerns due to the use of radioactive materials requiring strict handling protocols.
- Limited lifespan of radioactive isotopes necessitates periodic replacement.